Part
2 - Detailed Designs
part 1 - Initial Design Considerations

As mentioned in part 1 of this article series, the
Great Alaskan 25-28 was designed with the following
goals in mind:
- Seaworthy
- Efficient to Operate
- Suitable for slow-speed operation, e.g. sight-seeing,
camping, fishing, etcetera
- Larger than other similar boats yet still legal
to trailer without a special permit
For those that did not get a chance to read part
1 of this series, you will have missed some of the
philosophies of design that went into this boat. Since
it has been a couple of years since that last article,
each of the sections below will provide a short review
of what went into meeting the goals that I have listed
above along with some tutorials on the concepts underlying
the decisions made while designing this boat.
Seaworthiness and Stability
First and most important is the goal of seaworthiness.
Although there are many terms bandied about when it
comes to discussions of stability, e.g. initial stability,
ultimate stability, etcetera, there are really only
two primary types of stability (or stability factors)
that all boats are subject to. The first is weight
stability. If a boat’s vertical center of gravity
(VCG) is below, preferably well below, the vertical
center of buoyancy (VCB, the centroid of the submerged
volume) then the boat is said to have weight stability.
If the VCG is equivalent in height to the VCB, then
the boat has neutral weight stability. That means
the boat’s center of gravity isn’t going
to help, or hurt, a boat in righting itself once heeled.
If the VCG is above the VCB however, the boat has
negative stability and the boat’s center of
gravity is detrimental to the boat’s ability
to self-right when it heels.
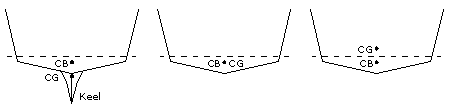
The hull on the left
has positive weight stability, the center has neutral,
and the right has negative weight stability. Boats
with negative weight stability depend on form stability
and hydrodynamics to overwhelm the negative stability.
Only displacement hulls such as sailboats and slow
cruisers have the opportunity to keep the VCG below
the VCB however, so planing hulls are out of luck
in this respect. Planing hulls must, since they ride
on top of the water, have a VCG above the VCB when
planing but the boat’s hydrodynamics and form
stability far overwhelm the negative weight stability.
When planing hulls are not planing however, a good
design goal is still to produce a VCG as low as possible
so at worst, it has only a minimal negative effect
on the boat’s ability to right itself when it
has been heeled over. The Great Alaskan utilizes lighter
construction up high, and heavier-than-required construction
low in the hull, and we recommend locating heavy components
such as fuel tanks in the belly of the boat in order
to keep the VCG as low as possible.
The second component to a boat’s stability
is form stability. Consider for a moment a cylinder
of Styrofoam floating on the water, and beside it,
a flat raft of Styrofoam that is wide versus its thickness.
The cylinder can easily be spun or rolled on the water
and it shows no tendency whatsoever to maintain any
particular orientation to the surface of the water.
The flat raft of Styrofoam however is tough to flip
over. You can push one edge down quite far and it
always self-rights when you let go. The difference
between the two chunks of Styrofoam is how far the
center of buoyancy moves to one side when the foam
is ‘heeled’ or rotate on the water. As
the cylinder spins, the center of submerged volume,
the CB, stays where it started. With the flat raft
of Styrofoam, the center of submerged volume, the
CB, moves far off to one side and exerts a higher
righting moment (or force.) In mathematical terms
you are observing the difference in the two pieces
of Styrofoam’s Transverse Metacentric Height
(GMt), a quantitative measure of the floating body’s
ability to self-right when heeled. The lesson here
is that wide boats with shallow deadrise (or even
flat-bottomed) self-right easier than boats that more
or less float like a cylinder in the water, e.g. heavy
deep-V hulls:
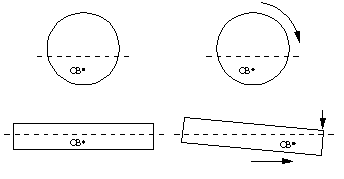
If the center of buoyancy does not move when a vessel
heels (top), then the vessel has zero form stability.
If the center of buoyancy moves to one side when a
vessel heels, then it does have form stability. Narrow
hulls with greater deadrise often have lowered form
stability, while wider hulls with lower deadrise most
often have greater form stability.
In summary, planing hulls without deep keels (rare!)
can not generally take advantage of weight stability,
but can take full advantage of form stability. Heavy
deep-V hulls must then have higher freeboard in order
to prevent down-flooding when waves or swells climb
up the side of the boat or when the boat is heeled
far over. But lighter weight, shallower deadrise,
hulls can get away with lower freeboard. In trade,
they must have a higher transverse metacentric height
(GMt) instead. GMt’s for heavier deep-V hulls
in the 24 to 28 foot category tend to run from 32”
to 44”. Don’t worry too much about what
the number of inches means, but note that higher inches
means a stiffer boat, one that rolls with the water
rather than resisting it. There is no magic number
that the GMt should be, but you should compare it
to what other boats in a similar class of boats have,
boats that are proven successful. If the GMt is too
low, the boat will roll late in comparison to when
the wave or swell rises under the boat (a sickly motion)
or will generate an extra-large roll now and then
(an odd whipping back and forth.) If the GMt is too
high, then the boat snap-rolls with the changing surface
of the water and is both uncomfortable to be in and
high mechanical stresses are placed on the boat instead.
When a GMt is selected as a target for a particular
design, it should be similar in size to the example
boats with which you wish to compare, but with an
adjustment up or down depending on whether you wish
to optimize the boat differently than the example
boats. The Great Alaskan has a GMt of 49” to
50-1/2”, depending on loading. This is slightly
higher than you see for heavy fiberglass boats and
the boat will roll with the water in a snappier fashion
as well. Noting that the number one reason for a boat
capsizing when drifting or moving slowly is the taking
of a wave over the side (or stern) of a boat, it is
important that boats designed for slow or drifting
usage are able to bob up and over waves rather than
allowing them to climb up the sides (or stern) of
the boat too highly. The goal is to be safe even if
not on plane.
There are a few other minor factors that have been
designed into the Great Alaskan in the name of seaworthiness
or safety at sea as well. The boat utilizes a semi-dory
sea skiff hull form which has a reasonably significant
amount of flare to the boat’s sides. This means
that the boat’s pounds per inch immersion (PPI)
dramatically increases as the boat is momentarily
pressed down into the water as the ocean around it
heaves (moves vertically upwards.) Having flared sides
also means the boat’s righting arm (GZ) increases
faster than you would see with a similar boat without
flared sides. The righting moment is the GZ times
the boat’s displacement, so (obviously, I hope)
the boat’s righting moment is more powerful
for any particular degree of heel than it would be
otherwise. The Great Alaskan’s “curve
of areas”, which represents the boat’s
fore and aft underwater volumes was designed to be
as balanced as possible for a planing hull. What this
really means is that the bow sections are as healthy
as possible without making the boat into a ‘pounder’,
and the aft sections are slightly reduced. The stern
of the Great Alaskan is a little narrower than the
amidships beam. This helps the boat respond properly
to both maneuvering efforts and to swells as the boat
runs down one and up the next. If a boat is too fine
in the bow, it will plow or dive into the next swell
and generate a sudden upward pitching moment in addition
to resisting appropriate steerage. Noting that the
local water speed in a wave is higher at the peak
than in the trough, that this occurring in a following
sea will tend to drive the stern to one side, a dangerous
situation called ‘broaching’ that can
lead to capsize as a boat is subjected to a large
swell or wave sideways. If a boat is more balanced
in its fore and aft sections, then this effect is
minimized. The Great Alaskan was also designed so
that its center of lateral resistance is not too far
aft of the center of buoyancy since this can also
result in a boat’s tendency to broach.
The final factor to consider is the boat’s
freeboard. The distance from the water surface to
the sheer, since this is how high water must go before
it can down-flood into the boat. Rather than utilize
a classic sheer line that dips lowest in the amidships
region, common in work boats, the Great Alaskan has
a sheer that rises constantly from stern to stem in
a sweeping arch. The boat is over 5 feet deep at the
bow and the amidships freeboard is higher than similar
vessels by 2 to 4 inches. The Prince Rupert version
of the design utilizes a 7” bulwark forward
and a 2” coaming aft that further increases
how far the boat can heel before down-flooding occurs.
Even without the coaming and bulwark though, the Great
Alaskan can heel 60 degrees before down-flooding occurs,
and the Prince Rupert model can heel several more.
This is 10 to 15 degrees more than what similar commercially-designed
boats can absorb.
Note: Some discussions concerning stability mention
terms such as initial stability and ultimate stability.
Ultimate stability has to do with the maximum number
of degrees of heel that a boat can absorb before either
down-flooding or negative stability occurs. Open-cockpit
planing hulls can not be effectively sealed up to
prevent down-flooding, so the point of negative stability
(the boat’s preference to remain upside down)
is never reached and down-flooding is instead the
limit. Initial stability is the boat’s resistance
to heeling the first 10 degrees or so. The chart below
shows the Great Alaskan’s righting moment versus
degree of heel and clearly demonstrates that the boat
has been designed to be more stable over this initial
‘comfort zone’ in heel angles:
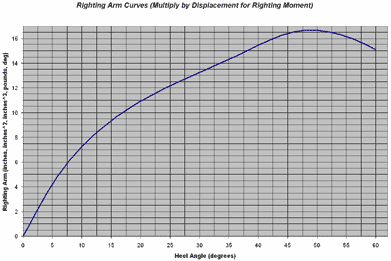
Notice: a) the curve
is steeper over the first 10 degrees, b) the righting
moment grows continuously clear up to 50 degrees of
heeling, and c) the boat can heel to 60 degrees before
down-flooding will occur (for the Newport …the
Prince Rupert can roll further before down-flooding).
This curve was generated in a free-float condition,
which means “as the real boat would perform”
rather than just by rotating the boat about its longitudinal
axis. See the full hydrostatics report at: https://www.glacierboats.com/hydrostatics.html
Efficiency
The number one factor that either hinders or helps
a boat to be efficient is the boat’s weight
or displacement. The second most important factor
is the hull’s overall resistance, whether planing
or operating in displacement mode or somewhere between.
The overall resistance is determined by factors such
as loading (pounds per square foot) and the form or
shape of the hull. The last of the factors worth listing
that can hurt efficiency is the parasitic resistance
of the finished boat. For example, things such as
surface rust and appendages rudders, outboards, or
other hardware that extend into the water increase
the boat’s parasitic resistance. Note: The term
‘residual resistance’ which you will see
in some literature refers to the resistance associated
with wave making (water pushed aside by the boat)
and should not be confused with the term “parasitic
resistance.”
The most efficient class of boats are slow displacement
boats. The second most efficient class of boats are
planing hulls. The third and least efficient of all
boat classes are the semi-displacement boats that
operate in a mode that is not quite on plane yet is
still beginning to lift out of the water enough to
not be in displacement mode either. (More info on
this topic below.)
Weight or Displacement:
Wood-composite or stitch-n-tape construction
weighs, on the average, about 40 pounds per cubic
foot of material. Some say 35 pounds, but my calculations
differ and with some wood selections I find that wood-composite
construction can actually reach densities as high
as 45 pounds per cubic foot. Aluminum weighs about
170 pounds per cubic foot and requires about half
the volume of materials as compared to wood-composite.
Four times heavier but half the volume means aluminum
boats of similar size weigh about twice as much as
a wood-composite boat. Polyester fiberglass layups
weigh about 100 pounds per cubic foot (but varies
quite a lot depending on total composite density and
core materials utilized in the layup.) Polyester fiberglass
boats may use more or less physical volume than wood-composite
constructed boats depending a lot upon what type of
cores and structure is utilized to produce the required
strength levels. Because polyester fiberglass is not
in itself very strong and must depend on additional
thickness, structure, and cores to produce the strength
required for a boat however, polyester boats do tend
to weigh quite a lot …often more than equivalent
aluminum boats, especially when comparing deep-V offshore
hulls.
The bottom line here is that the wood-composite method
of boat building is by far the lightest way of building
mid- to large-sized boats and has the most potential
for producing a high-efficiency hull in this size
range and displacement capability. The primary reason
that commercial makers don’t use this method
for building their boats is cost, and the primary
factor of which are the required man-hours, not the
cost of materials. You can’t spray a wood-composite
boat into a mold, and forming composite seams with
fiberglass and epoxy results in fewer inches of seam
produced per hour of work compared to standard weld
rates. But there is no reason that a home builder
(who works for ‘free’) cannot take advantage
of what wood-composite construction has to offer.
Because efficiency is of prime importance to me as
a designer, especially in light of increasing oil
prices, wood-composite is my natural go-to first choice
when I’m deciding what material and building
method that I’d like to use.
Hull Form v. Resistance:
The overall resistance of a planing hull is most
directly a result of the boat’s deadrise, change
in deadrise from aft to fore, and its fineness of
entry. Deep-V hulls have a higher prismatic coefficient
of lift in the forward sections that can result in
a more aggressive pitching motion (upwards) when the
hull enters larger waves. You definitely do not want
a boat that has too fine of an entry versus the width
of the rest of the boat’s hull, and this is
doubly true for lighter boats that have a lower longitudinal
moment of inertia (the tendency to resist pitching
as a result of the boat’s weight and fore/aft
distribution of weights.) On the other hand, Flat
bottomed boats tend to be comfortable only on calm
water since they tend to pound when in rougher conditions.
Wider boats are more efficient on calm water, yet
narrower boats that slice through waves more nicely
tend to be more efficient in rough water. For any
particular boat, the designer can optimize the boat’s
average resistance by making assumptions on the speeds
and sea states that the boat is intended to operate
in. Failure to do this will result in a boat with
disappointing performance. The Great Alaskan’s
26-1/2 degree half angle of entry, 14-1/2 degree amidships
deadrise, and the Savitsky waterline ratio (at-rest
waterline beam divided by mean waterline length) were
selected to be a best compromise for a boat that is
intended to operate in average choppy offshore conditions
at speeds averaging about 19-20 knots. The Great Alaskan’s
5” wide chine flats are intended to be a best
compromise between having a lot of horizontal lifting
surface, yet not so much that the boat pounds.
The Great Alaskan also has a highly prismatic, monohedron,
type hull below the waterline. What this means is
that there is not much change in deadrise from the
stern to the amidships region where most of the planing
lift is generated. There is only about 1-1/2 degrees
difference from stern to amidships. Other hulls tend
to have 4 to 6 degrees difference in deadrise and
consequently waste more energy sending water out sideways
from the boat rather than downwards. Water sent sideways
means that the boat spent energy in a direction that
does not help the boat plane, e.g. lost efficiency.
Since the Great Alaskan is optimized for 19-21 knots
(top speeds in the upper-30 knot range), this hull
form works fine for the speeds that most people are
willing to travel when in offshore conditions. The
Great Alaskan’s length of 25 to 28 feet allows
the transition from the entry deadrise to the amidships
deadrise to take place over a longer span than what
would be available on shorter boats, and this is taken
advantage of in this design to allow both a reasonably
fine entry yet still maintain modest-deadrise prismatic
hull from amidships aft for efficiency. I would not,
for example, use this hull form on a boat only 22
feet long intended for similar speeds because the
boat would simply not be long enough for the required
rate of transition in the bow. But I certainly would
when considering design parameters for a longer boat
such as the Great Alaskan.
Finally, all V-hull boats tend to allow water to
travel up the sides of the hull, turning into spray
that blows into the boat as it comes off the sides
of the boat. All V-hulls, if you want a dry boat that
is, should include some type of water rejection that
prevents this upward climbing of water on the boat’s
sides. The heavier the boat and the higher the deadrise
angles, the worse these problems become. Water rejection
by the Great Alaskan is accomplished by the combination
of the 5” wide chine flats, the auxiliary spray
rails along the chines forward that actually drive
the sheet of water coming off the bow back downwards,
and by the main spray rails along the sides. The models
of the Great Alaskan that have larger pilot houses
add additional water rejection capability by draining
water that does land on top of the boat to the sides,
and on the Prince Rupert, by cockpit coaming that
extends aft to alongside the drywell.
Accommodations and Boat Size
Trailers:
One of the goals for the Great Alaskan was to provide
the maximum amount of room inside the boat as possible,
yet still stay within federal limits for trailers
on the United States interstate highways. According
to federal law, all interstate highways have to allow
up to 8’6” wide trailers or loads plus
reasonable access to and from the interstate. Reasonable
access includes, for a boat, the roads to and from
waterways and storage facilities, whether it is your
home or otherwise. The Great Alaskan, when built exactly
to specifications, turns out 8’5” wide
including the rubrails along the sheer. This allows
a bit of room for error and/or some flexibility on
the part of the user in choosing the type and style
of the sheer line rubrails.
Another design constraint that must considered when
designing a boat of this size is whether or not it
will fit onto standard, non-customized, trailers.
Nobody wants to spend money on custom trailers if
they don’t have to. For this boat, any tandem-axle
trailer with a maximum weight capability of 5000 to
7000 pounds and has 82” or more room between
fenders will work for the Great Alaskan, noting that
a typical trip will result in around 3800 pounds (boat,
motors, gear, crew, fuel, etcetera) and the maximum
capacity of the Great Alaskan is around 6000 pounds.
For this boat, some manufacturers may require you
to order a longer tongue on the trailer, but that’s
fairly normal on trailer orders anyway and it is a
low-cost change to ask for. The interior construction
has already been designed to allow the use of your
choice of roller or bunk type trailers. Brake requirements
vary from state to state, but all trailers in this
size category have all brake options available. Fortunately
the Great Alaskan’s waterline beam, which would
normally sit just about even with the tops of the
fenders when the boat is on the trailer, is 79”
wide. It should not be hard or expensive to find a
trailer for this boat.
Accommodations:
As far as accommodations go, the Great Alaskan is
superior to the commercial boats that I have reviewed
and has more room throughout. Part of the reason for
this is that aluminum boats are generally not designed
to be campers or cruisers, and polyester fiberglass
boats require additional thickness and structure in
order to make them strong enough (as described previously.)
While fiberglass boats may commonly be designed for
camping and cruising, they invariably have several
inches less space inside them when compared to a wood-composite
boat such as the Great Alaskan. As an example, consider
the Great Alaskan’s cuddy. The cuddy compartment
has nearly 7’ sized bunks in it and enough room
to design-in a drop-down table (queen-sized bed when
lowered, moderately-sized bedroom dinette when raised.)
And inside the pilot house, the boat has a full 6’3”
of headroom above a deck that runs flush from transom
to cuddy. There is room for a stand-up head and shower
should you desire, a galley, dinette, and seating.
The plans package takes an approach that shows you
basic methods of building all the above, including
framing and interior support, and then gives example
layouts that you may wish to use. Or, you can design
your own. Whatever you do, I am willing to assist
with final placement of heavy items that will help
your boat trim properly at the dock and to perform
properly when not. I have full center-of-gravity spreadsheets
already produced for the boat (required for design
anyway) and can easily model your trim, hydrostatics,
and hydrodynamics if you provide me with information
on what you wish to put in the boat and where.
Typical accommodations
for the Newport or Prince Rupert. In order to provide
the most flexibility possible in arrangements and/or
cuddy or pilot house sizes, the plans provide instructions
for building all 3 models of the boat in sizes ranging
from 25 to 28 feet long and also provide acceptable
ranges of locations for the primary bulkheads (fore/aft
house and cuddy bulkhead locations.)
Current and
Future Status
As of the date of this article, there are 6 builders
actively building and I expect that the first hull
will launch sometime in mid-2008. Builders currently
exist in Alaska, Washington, California, Nevada, Virginia,
and Florida. Printed and download-only plans are available
both here at Duckworks Magazine and at our own web
site at https://www.glacierboats.com.
(But say thank you to Chuck for publishing this article
for me by buying your plans through him if you can.)
Plans include 200+ pages in construction manuals (2
parts) and 29 drawings designed to print on 11x17
(Arch-B) paper. All documents are in pdf format and
those that opt for printed plans are given access
to the online repository of the pdf versions of the
documents as well. Support will be provided via email
and evening/weekend phone access whenever I’m
available. If it seems like a good idea then I may
set up a forum-type environment for builders to interact
as well. The web site will be updated with photos
from all builders as they are provided to me of course,
and after the snow returns, I will be putting together
some better 3D photo-realistic renderings that will
also be made available at the web site. If you are
interested in having your name added to the ‘interested
parties’ distribution list, just email me (https://www.glacierboats.com/contact.html)
and let me know.
Brian
back
to part 1 - Initial Design Considerations
Plans
for Great Alaskan are available at Duckworks

|